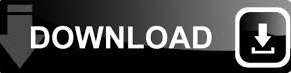

This review will address new concepts and strategies through which the rational design of CPPs can serve to enhance the efficiency of internalization and regulate the kinetics of this process. However, the challenge is to introduce this property in a controlled and programmable manner through rational design. The folding ability of CPPs to form α-helices and β-sheets increases their efficiency to penetrate cells, as described in more detail in Section 4.2. In addition, the role of properties such as sequence length and conformation in internalization is equally as important as the accumulation of positive charge. Moreover, internalization can vary in function of overall amphipathicity and polycationic nature. Charge and amphipathicity are the two main structural parameters taken into consideration when designing CPPs. Įxtensive literature is available on the structure–activity relationship of primary CPPs and the folding properties of amphipathic peptides. Statistical graphic representations depicting the distribution of cell-penetrating peptides (CPPs) reported in the literature (based on 1855 entries, of which 1699 are unique peptides) on the basis of the following: ( a) linear and cyclic structure ( b) type of cargo delivered and ( c) chirality (taken from the CPP 2.0 database), taken from reference. Moreover, the internalization efficiency and stability of all D-stereoisomers have been reported alongside CPP sequences composed of L- and D- combinations ( Figure 1c). The main body of research on CPPs focuses on synthetic peptides (54.8%) based on L-amino acids (84.3%) ( Figure 1c). In addition, proteins (9%), nanoparticles (7.8%) and peptide therapeutics (4.7%) are other cargoes of interest ( Figure 1b). The major cargo of biomedical relevance is nucleic acids (16%). Interestingly, the statistics section of the CPP database site ( Figure 1) reveals that there are 1699 unique CPP sequences and that most are linear CPPs (94.5%) ( Figure 1a) used for the delivery of fluorophores (58.4%) ( Figure 1b). Ī CPP database, established in 2012 and now updated to CPP version 2.0, is a useful source of information. Positively and negatively charged, amphipathic (primary or secondary) and non-amphipathic CPPs are used today in in vitro and in vivo studies. Inspired by these molecules and focused mainly on positively charged sequences, many CPPs with differences in charge, polarity and/or structure have been discovered and/or designed. Discovered in the 1990s, the first reported examples of CPPs were the Tat peptide (positions 48–60, derived from the transcription protein of HIV-1) and penetratin (derived from the amphiphilic Drosophila Antennapedia homeodomain). An alternative terminology, the protein transduction domain (PTD), was used initially to describe such peptides as the first examples of CPPs were based on natural peptides derived from protein fragments. Therefore, the transition of CPPs to a clinical setting remains a challenge also due to the concomitant involvement of various internalization routes and heterogeneity of cells used in the in vitro studies.Ĭell-penetrating peptides (CPPs) are short peptides (<30 amino acids long) that are able to penetrate biological membranes and drive the internalization of a bioactive cargo in cells. This led to increased design complexity of new CPPs that does not always result in greater CPP activity. The application of distinct design strategies to known physico-chemical properties of CPPs offers the opportunity to improve their penetration efficiency and/or internalization kinetics. In this review, a variety of CPP designs will be described, including linear and flexible, positively charged and often amphipathic CPPs, and more rigid versions comprising cyclic, stapled, or dimeric and/or multivalent, self-assembled peptides or peptido-mimetics. Following these nature-inspired examples, a number of rationally designed CPPs has been developed. The discovery of cell-penetrating peptides (CPPs) and the early design approaches through mimicking the natural penetration domains used by viruses have led to greater efficiency of intracellular delivery. Thus, compounds that efficiently cross cell membranes are the key to improving the therapeutic value and on-target specificity of non-permeable drugs. Efficient intracellular drug delivery and target specificity are often hampered by the presence of biological barriers.
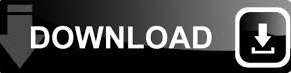